First Stand-Alone, Flexible, Fully Organic Bioelectronic Device Paves Way for Soft and Safe Medical Implants
Posted on 13 Jul 2023
Amidst the significant advancements being made in medical care, researchers are also finding out that adopting individualized approaches can further enhance the efficacy of these treatments. As a result, clinicians require approaches that can continuously monitor physiological signals as well as personalize responsive delivery of therapeutics. Implantable bioelectronic devices play a vital role in such treatments, although their widespread adoption has been hampered by various challenges such as the requirement of specialized components for signal acquisition, processing, data transmission, and powering. Until now, developing an implantable device with such capabilities has involved the use of various rigid and non-biocompatible components that can cause tissue disruption and patient discomfort. Such devices need to be biocompatible, flexible, and stable for remaining inside the body over the long term while simultaneously being fast and sensitive for recording rapid, low-amplitude biosignals and still transmitting data required for external analysis.
Researchers at Columbia Engineering (New York, NY, USA) have now developed the first ever stand-alone, conformable, completely organic bioelectronic device that is capable of acquiring and transmitting neurophysiologic brain signals, as well as providing the power required for its operation. Approximately 100 times smaller than a human hair, the device is built on an organic transistor architecture that integrates a vertical channel and a miniaturized water conduit with long-term stability, high electrical performance, and low-voltage operation to avoid any damage to biological tissue. Researchers and clinicians were well aware of the need for transistors that simultaneously featured low voltage of operation, biocompatibility, performance stability, conformability for in vivo operation; and high electrical performance, including fast temporal response, high transconductance, and crosstalk-free operation. Despite silicon-based transistors being the most established technologies, they are hardly a perfect solution due to their hard, rigid properties, and inability to establish a highly efficient ion interface with the body.
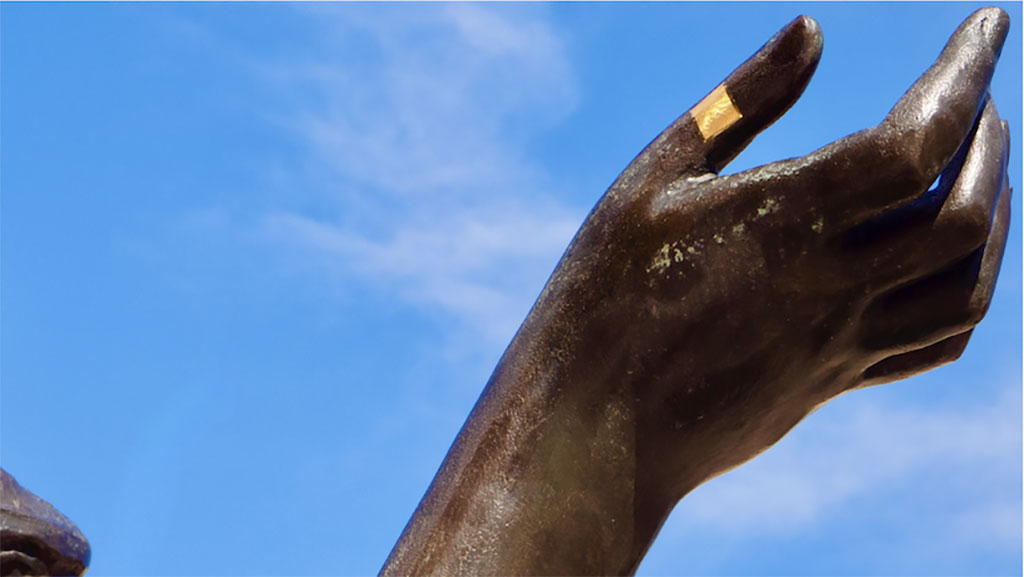
To address these challenges, the researchers introduced a scalable, self-contained, sub-micron IGT (internal-ion-gated organic electrochemical transistor) architecture, the vIGT. The team incorporated a vertical channel arrangement that increases the intrinsic speed of the IGT architecture by optimizing channel geometry and enabling a high-density arrangement of transistors next to each other – 155,000 transistors per centimeter square. The vIGTs are made of biocompatible materials available in the market that do not need encapsulation in biological environments and are not affected by exposure to water or ions. The channel’s composite material can be reproducibly manufactured in large quantities and is solution-processible, allowing it to be used in a broad range of fabrication processes. Additionally, they are flexible and compatible with integration into a wide variety of conformable plastic substrates. They also offer long-term stability, low inter-transistor crosstalk, and high-density integration capacity, enabling the fabrication of efficient integrated circuits.
Using advanced nanofabrication techniques to miniaturize and densify these transistors at submicro-meter scales, the researchers increased the speed of operation even further. In order to develop the architecture, the team was required to first develop an understanding of the challenges in diagnosing and treating patients suffering from neurological disorders such as epilepsy, along with the methodologies presently in use. By combining high-speed, flexibility, and low-voltage operation, the transistors can be used for neural signal recording and data transmission, as well as for powering the device, resulting in a fully conformable implant. Using this feature, the researchers demonstrated fully soft and confirmable implants which can record and transmit high-resolution neural activity from outside, on the surface of the brain, as well as inside, deep within the brain. The researchers will now work with neurosurgeons to validate the capabilities of vIGT-based implants in the operating room and aim to develop soft and safe implants capable of detecting and identifying different pathological brain waves created by neurological disorders.
“This work will potentially open a wide range of translational opportunities and make medical implants accessible to a large patient demographic who are traditionally not qualified for implantable devices due to the complexity and high risks of such procedures,” said Jennifer Gelinas, assistant professor of neurology, electrical and biomedical engineering and director of the Epilepsy and Cognition Lab.
Related Links:
Columbia Engineering