Miniaturized Fiber Photoacoustic Spectrometer Enables Real-Time Intravascular Blood Gas Monitoring
Posted on 06 Jan 2025
Miniaturized spectroscopy systems capable of detecting trace concentrations at the parts-per-billion (ppb) level are crucial for various applications, including biomedical diagnostics. However, traditional bench-top spectroscopy systems are too large, complex, and impractical for use in confined spaces. Additionally, conventional laser spectroscopy techniques rely on bulky components such as light sources, mirrors, detectors, and gas cells to detect light absorption or scattering by a sample. This makes them unsuitable for situations requiring minimal invasiveness, such as intravascular diagnosis. To address these limitations, researchers have developed a novel miniaturized all-fiber photoacoustic spectrometer (FPAS), designed for detecting trace gases at the ppb level and analyzing nanoliter-sized samples with millisecond response times, making it ideal for continuous intravascular gas analysis.
In a study published in Advanced Photonics, researchers at Jinan University (Guangzhou, China) introduced the FPAS, which is designed to detect trace gases at the ppb level while analyzing nanoliter-sized samples with fast response times. The researchers focused on overcoming the challenge of reducing the size of traditional photoacoustic spectrometers to a microscale while maintaining high sensing performance, particularly for applications like intravascular diagnosis and lithium battery health monitoring, which require minimal invasiveness. While current laser spectroscopy systems, typically used in open-path configurations, face challenges in scaling down sensitivity due to the device's footprint, the FPAS operates using photoacoustic spectroscopy (PAS). PAS detects sound waves generated by gas molecules when excited by modulated light.
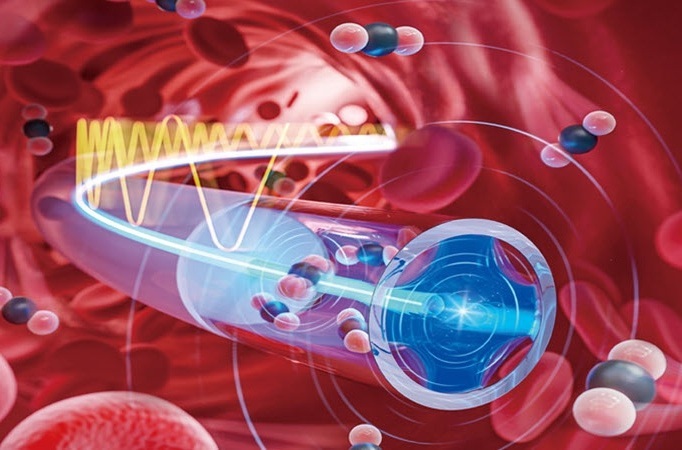
Unlike traditional PAS systems that use bulky resonant gas cells for acoustic amplification or large microphones for increased acoustic sensitivity, the all-fiber FPAS integrates a laser-patterned elastic membrane into a single optical fiber tip along with a silica capillary to create a microscale Fabry–Perot (F–P) cavity. The silica cavity serves as a sound-hard boundary, efficiently trapping and accumulating the acoustic waves generated by the gas molecules toward the flexible membrane. This local acoustic amplification compensates for sensitivity loss caused by the reduced membrane diameter, resulting in a size-independent photoacoustic response. Moreover, both the pump and probe light beams are delivered through the same fiber for excitation and detection of the photoacoustic signal, eliminating the need for bulky free-space optics.
With the F–P cavity measuring only 60 micrometers in length and 125 micrometers in diameter, the system is incredibly compact. Despite its small size, it achieves an acetylene gas detection limit as low as 9 ppb, nearly matching the sensitivity of larger traditional laboratory spectrometers. The short cavity length also enables rapid measurements, with response times as fast as 18 milliseconds, which is 2 to 3 orders of magnitude faster than conventional photoacoustic spectroscopy systems. The researchers successfully used the FPAS to monitor real-time carbon dioxide (CO2) concentrations in flowing gas, detect fermentation in yeast solutions with sample volumes as small as 100 nanoliters, and track dissolved CO2 levels in rat blood vessels in vivo by inserting the FPAS into the tail vein via a syringe.
The spectrometer effectively measured CO2 levels under hypoxic (low oxygen) and hypercapnic (high CO2) conditions, demonstrating its potential for real-time intravascular blood gas monitoring without the need for blood sample collection. Additionally, the optical fiber can be easily connected to a low-cost distributed-feedback laser source and integrated into existing fiber-optic networks, making the system a cost-effective, compact, and flexible solution for spectroscopy. With its small size, high sensitivity, and low sample volume requirement, this miniaturized spectrometer offers laboratory-level precision in a microscale probe format. It holds potential for applications such as continuous intravascular blood gas monitoring, minimally invasive health assessments of lithium-ion batteries, and remote detection of explosive gas leaks in confined spaces.